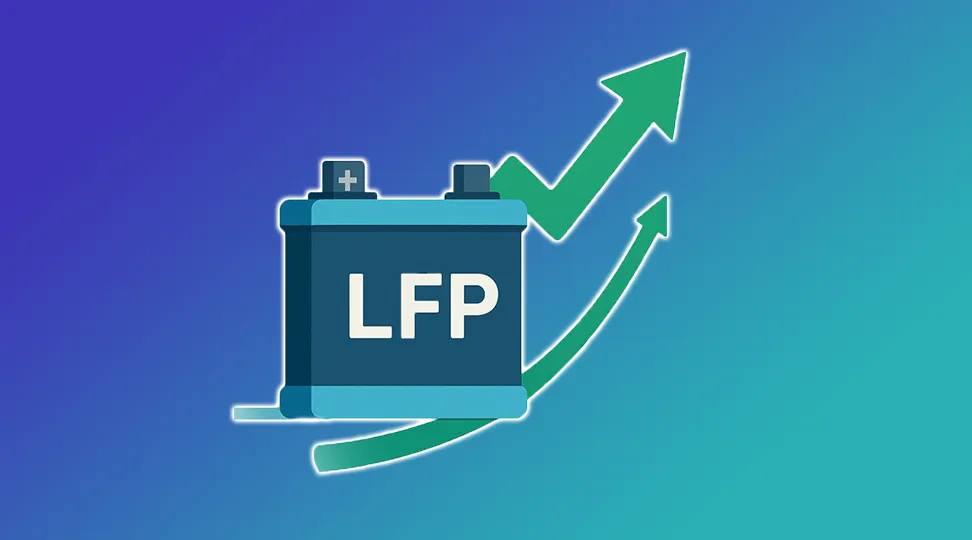
A Brief History Of LFP Batteries
Table of Contents
LFP batteries have become the dominant battery in global EV production and have recently sharply fallen in price to a stunningly low $115/kwh.
What Makes A Given Battery A Given Chemistry?
Like other batteries, lithium-ion batteries have two electrodes, a cathode and an anode. The anode, which is the positive end in these batteries, is generally just graphite—nothing too special there. The cathode is where all the magic happens: that’s where the chemistry lives. It’s also the most expensive part of the battery, making up about 70% of the total value, and it’s the main distinguishing factor between different types of batteries.
Two Main Categories: Ternary vs. LFP
There are two main categories of lithium-ion batteries: ternary batteries (made of three elements) and LFP batteries. Ternary batteries mix three different chemicals—either nickel, manganese, cobalt (NMC) or nickel, cobalt, aluminum (NCA)—in varying ratios. These batteries offer the highest energy density but use rare materials, making them more expensive. They’re also sourced from geopolitically tense regions and, due to their high energy density, have a greater risk of thermal runaway.
LFP batteries use two different chemicals on the cathode—iron and phosphorus. There’s no nickel or cobalt, and iron and phosphorus are far more common worldwide. This makes LFP batteries much cheaper to produce and gives them a major advantage in cycle life: while NMC batteries retain about 80% of their capacity after 500–800 cycles, LFP batteries can exceed 2,000 cycles without significant degradation. If you’re looking to squeeze even more longevity out of your cells, check out our guide on How To Get More Cycles Out Of Lithium Ion Cells.
What Is The Downside Of An LFP Battery?
The big drawback of LFP batteries is their lower energy density—around 90–150 Wh/kg compared to 260–300 Wh/kg for NMC and NCA. Two same-sized batteries, one LFP and one ternary, will store more energy if they use ternary chemistry. However, innovations in LFP chemistry, cell design, and pack integration have begun to narrow this gap. For a side-by-side comparison of these chemistries, see our full breakdown in NMC Vs. LifePO4 Which Is Best. It’s also important to remember that each chemistry (NMC, NCA, LTO, LFP) has many variations, and pack-level choices can significantly affect performance.
Who Invented LFP Batteries?
John B. Goodenough—yes, that’s his real name—first proposed and patented iron phosphate as a lithium-ion cathode material in the mid-1980s. His LFP chemistry promised better safety and cycle life but suffered from low electrical conductivity and poor energy density (around 90 Wh/kg), limiting its early appeal for electric vehicles.
Why Are LFP Batteries So Cheap Now?
The remarkable affordability of LFP batteries today is the result of many years of advances in materials science, strategic patent licensing and consolidation, and targeted policy incentives that drove early large‐scale adoption. By pooling and licensing key conductivity-enhancing patents, deploying subsidies to jump-start fleet electrification, and then allowing market forces to favor LFP’s inherent safety and longevity as subsidy support for higher-density chemistries waned, manufacturers achieved the economies of scale necessary to push prices sharply downward.
Conductivity Boosts and Patent Pools
In the 2000s, Canadian and European companies formed institutions to develop carbon-coating processes and related patents to enhance LFP conductivity. These patents were consolidated into a Swiss licensing consortium. In the 2010s, Chinese battery firms secured royalty-free domestic rights to these key LFP patents, whereas others worldwide had to pay licensing fees. Initially, Chinese firms could only use these patents domestically.
China’s TVTC Policy and Early Adoption
In 2009, China launched the TVTC policy (Thousands of Vehicles, Tens of Cities), a subsidy program encouraging local governments to deploy buses, taxis, and government vehicles equipped with LFP batteries. BYD then introduced LFP packs in its eBus and early hybrids, establishing domestic leadership. Outside China, most OEMs still preferred NMC or NCA for their higher energy density.
Shifting Subsidies and Market Share
Between 2016 and 2019, China shifted subsidies to favor EVs based on performance metrics (charge rate, range), prompting OEMs to chase higher-energy-density ternary chemistries. LFP’s share of new installations in China dropped from about 70% to 30% by mid-2019. Then, mid-2019, China halved its cash subsidies and continued annual cuts, narrowing the cost gap between ternary and LFP batteries. With subsidies rolling back, market forces favored LFP for its safety, cycle life, and lower cost—provided sufficient pack volume could compensate for lower energy density.
Blade Cells and Cell-to-Pack Innovation
In March 2020, BYD unveiled its Blade cells: ultra-thin prismatic LFP modules that eliminate intermediate modules in pack assembly. Traditional EV packs require cells → modules → packs; skipping the module step increases energy density at the pack level. BYD’s Blade-equipped Han EV achieves a 605 km range—about 85% of NMC density—while offering lower cost, better cycle life, and improved safety. Other Chinese OEMs swiftly adopted cell-to-pack (CTP) LFP technology, and by 2021 LFP pack volumes in China overtook NMC. By 2023, LFP accounted for 70% of EV installations in China.
Patent Expiry, Raw Materials, and Price Declines
By 2022, core LFP patents began to expire, but China’s industrial base and supply-chain expertise were already dominant. New lithium sources in Australia, Chile, and Argentina came online, and by 2024 iron-ore prices fell ~15% due to a Chinese steel slowdown. LFP pack prices dropped another 20% to around US $115/kWh—making Chinese LFP packs the cheapest globally, even after import tariffs of 25–120%. In 2021, BYD began selling Blade batteries to Toyota, Ford, and others, sparking a price war among battery suppliers.
Stationary Storage and Emerging Chemistries
LFP’s cost and safety advantages have fueled a boom in grid-scale and home energy storage. Its lower energy density matters less for stationary applications. Innovations continue: adding manganese to create LMFP (a ternary lithium-iron-based cathode) boosts energy density, and novel two-step firing processes improve particle uniformity and conductivity.
Conclusion
LFP cell costs are destined to continue declining, and energy density gains may soon challenge NMC’s dominance. What began as an American-engineered, safe but low-energy chemistry has—through Chinese licensing, policy, and technological innovation—become one of the world’s lowest-cost and most promising lithium-ion battery technologies. We hope this article helped you learn more about LFP chemistry and lithium ion batteries in general. Thanks for reading!