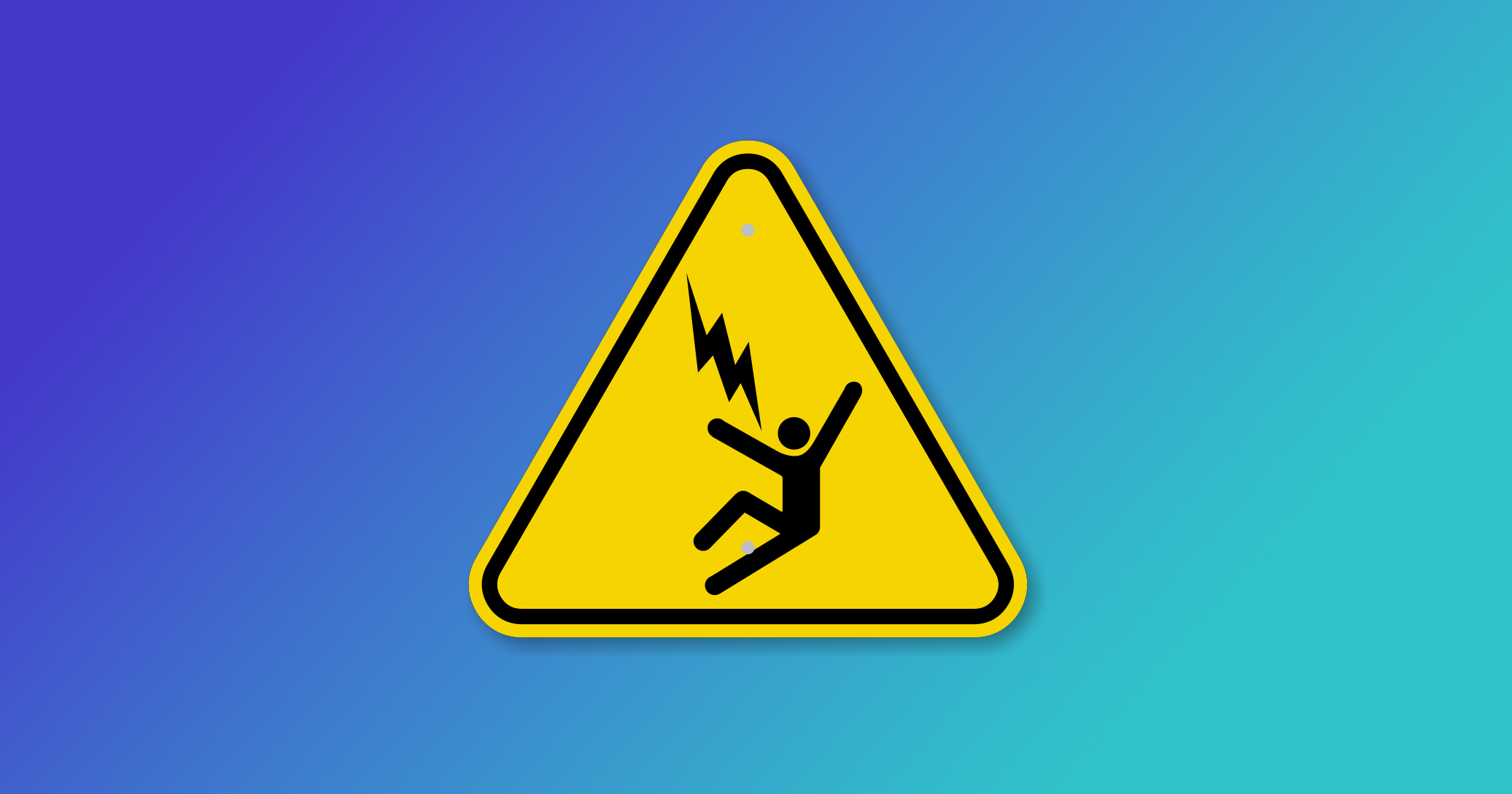
Is It The Voltage That Kills You Or The Current
Table of Contents
So, is it the voltage that kills you or is it the current? The age-old question—endlessly debated for well over a century.
Voltage can be thought of as the pressure of the electricity because voltage is literally the energy per unit charge of the electricity. Resistance is the amount in which a given material will resist the flow of that electricity, and current is the rate at which that electricity ends up flowing considering the amount of energy it had in the first place and how difficult it is for that energy to move through a given material. In this article, we will explain in depth what voltage, current, and resistance are. We will also explain how those things combine to form power. This might be somewhat of a controversial article, but let's get started.
What is Voltage?
Voltage is, quite literally, the measure of the potential energy per charge between two points. Put simply, voltage is the ability for a given material to create electricity. In order for electricity to happen, you have to have two things that have a difference in charge come into contact. Once that happens, that creates a voltage at that moment—that is when voltage is created.
A power supply creates a voltage like this. It uses various devices, components, and techniques to increase the charge on one side of the circuit while keeping the charge on the other side at a particular reference point. This difference in charge creates the voltage. Voltage only exists when there is a difference; if there is no difference in charge, there is no voltage.
Humans are really smart, so we can make all kinds of devices and combine all kinds of materials to control the charge of a particular thing. We can do that so well that we can control the charge between two particular things so precisely that we can produce exactly 12 volts of difference. Whenever you refer to voltage, you're really referring to how many volts of difference there are—because voltage is, by definition, a difference. If there's no difference, there is no voltage.
A battery creates a voltage like this. We create two different materials that, by their very nature, have two different levels of charge. We keep those materials separated so that they don't come into contact inside the battery. Then, we connect conductors to the outside of the battery so we have a positive and negative terminal.
What is Resistance?
This one's pretty easy to conceptualize, because resistance literally is just that—it is the resistance to the voltage, or how much it's going to lower the voltage. Resistance is the property of the material that defines how much the input voltage will be different from the output voltage. And one might interject and say, well, actually, a resistor regulates the flow of current in a circuit. Right, but how does it do that? If you are interested in resistance, then check out our article on resistance and how it affects a battery's performance
How Does A Resistor Limit Current?
A resistor affects the current flow by creating a voltage drop across itself, so it is lowering the voltage along the path of the circuit. Here is how it happens:
- In a circuit, with a voltage source (like a battery), the total voltage from the source remains constant (say 12V).
- When current flows through a resistor, it creates a voltage drop across that resistor according to Ohm's Law (V = IR).
- This voltage drop means that the electric potential decreases as current moves through the resistor - so yes, the resistor is effectively "lowering the voltage" at points after it in the circuit.
- The resistor limits current flow because the voltage drop it creates must obey Ohm's Law. Since the voltage source is fixed, the current must adjust according to I = V/R.
For example, in a simple circuit with a 12V battery and a 4Ω resistor, the current will be 3A because I = V/R = 12V/4Ω = 3A. The resistor creates a 12V drop across itself, and this voltage drop is what limits the current to 3A.
So, resistors do work by creating a voltage drop, which directly limits the current flow. In practical terms, we can absolutely think of resistors as lowering the voltage along the path of current flow.
The difference between the input voltage and the output voltage of a resistor is directly related to the rate at which the voltage is moving through the resistor. So if the voltage is moving through the resistor twice as fast, then it will produce twice as much voltage drop.
Imagine a fluid that an object is entering at a given speed, and it's attempting to pass through this fluid. The speed when exiting this fluid will be lower than the speed at the time of entering the fluid. If resistance is the fluid, then we can think of resistance as a type of material that, the faster you try to move through it, the more your exit speed will be reduced. Traditionally, we might think of a bullet going through a block of ballistics gel—in that situation, the more speed you have going in, the more speed you're going to have going out, even though the speed will always be lower. But in this situation, resistance has the properties such that as you move through it faster, its ability to slow you down increases instead of decreases.
Electronics Work At The Quantum Level
I know that sounds really strange, but that's okay because we are literally dealing with the quantum world here. The things that we are describing with volts, current, amps, ohms, resistance—all of that is just our way to classically describe a quantum thing. Quantum things don't have to obey classical physics.
For example, if we have a certain amount of balls in a given amount of space, we can absolutely predict with a very high degree of accuracy exactly where those balls are because we can measure them. But electrons can't be measured like that. We can only predict with a certain degree of accuracy the probability of their position at any given point. We can't even say with certainty that an electron is not two feet from its nucleus. All we can say with certainty is that it's 0.0001% likely to be there, so basically unlikely to be there.
And as you get closer and closer to where they actually are, the probability that they are there increases to almost, but never, 100%. This doesn't make classical sense, but it makes quantum sense. So we don't really have to understand why these things are happening; we just have to understand that it's true that they are happening, and we have to be able to relate how it will happen and what those effects will be with mathematical certainty. We don't have to be right about it, it just has to work.
What is Current?
Current is the rate that the electricity moves through the circuit. It is the rate in which electric charge flows. So, consider a two-ohm load with a 12-volt circuit. A circuit by definition connects back around to itself—that's why it's called a circuit. If we increase the charge on one side of the circuit to whatever level is required to produce 12 volts of difference when connected to the other side of the circuit, and put a two-ohm load in series with that, electric charge will flow. And the rate in which it flows, we measure in amps.
Ultimately, power supplies generate power that can be used because they maintain the difference between the high side and the low side of their outputs, and they are rated to maintain that difference up to a certain amount of speed. So, we can follow the entire path to see exactly when current is created. You either have a power supply that has active components or a battery that has material properties that produce two components that have different charges. When those two components come into contact, a voltage is created up to a particular maximum voltage. The actual voltage that's created depends on not just the resistance of the contact, but the resistance of the inside of the power supply or battery and every other part of the circuit—the wires, the connectors, the solder joints, the welds, the crimps; it all adds up.
That means we can't just consider the resistance of the load, which is two ohms. We also have to consider the sum total resistance of everything else. So, let's just say, for simplicity's sake, that the entire power supply and circuit and everything is 140 milliohms. We add that to our two-ohm figure. Current is the measure of the end result speed of the electricity moving through a given material. It's easy to find the speed because all you have to do is divide the voltage by the resistance.
If we take 12 volts and divide that by 2.14, we get 5.61. We know the speed of the electricity moving at any point throughout the circuit is 5.61 amps. The amount of power that happens as a result of that can be calculated by multiplying that speed by the voltage drop over a given section of the circuit. That comes out to 62.94 watts—that's the power for the entire circuit. But because only around 0.79 volts of that total voltage drop happens outside of the load, only a very small portion of the power is dissipated there. The overwhelming majority of the power goes to where there is an 11.22-volt drop across that two-ohm resistor, the load.
So, current itself, while it's very important, is just the end result of how electricity moves through resistance. With a given voltage and a given resistance, the energy will be able to move through that circuit at a given speed. If it's 12 volts and 6 ohms, the speed will be 2 amps. If it's 5 volts and 2.5 ohms, the speed will also be 2 amps. If it's 100 volts and 1,000 ohms, the speed will be 0.1 amps. Multiply the speed by the voltage, and you get the power. That's how the current is the same through the wires, connectors, and everything else, but most of the power isn't going there because the voltage drop in that area is lower, because the resistance is lower.
What Kills You? The Volts or the Amps?
So, what kills you? The volts or the amps? The answer is either or, or maybe both. I mean, you could also say it's the resistance that kills you; you could also say it's the charge that kills you. We could trace it all the way back through the conservation of energy, matter, momentum, and all of that stuff, and we can say the big bang is what really killed you. But the order of operations is important. The voltage definitely happens first, and the amps are just the speed that that voltage is happening.
So, what kills you? The bullet or the speed at which the bullet made contact with you? Technically, both of those things are true. If a much lighter thing hit you at the same speed, you wouldn't die. And if a much heavier thing hit you at a slower speed, you would die.
So, when it comes to what kills you—the volts or the current—the current is the one actually doing the killing. But if you don't have enough voltage, there will not be enough current to harm you because of the resistance of the human body. Another thing to consider is the fact that, ultimately, the voltage is the star of the show. It's the voltage that may or may not be limited enough by the resistance to kill you or not, and the current is just the end result—the speed of the electricity moving through that resistance. And you have to multiply that by the voltage anyway to get enough watts to kill you. So, I feel like it's the voltage that kills you.